Why consider Shared Spectrum for Private 5G and 4G LTE networks?
Shared spectrum solutions for 4G and 5G enable the use of the same spectrum range in a single geographic area by more than one organisation. This is different than Unlicensed Spectrum which is uncontrolled and generally suffers interference. Shared Spectrum is licensed and therefore Private 5G can operate in deterministic manner.
Here is a snapshot for 2020: recent examples of spectrum initiatives to private mobile networking are outlined (nonexhaustive list).

Which countries are planning Shared Spectrum?
Australia: In August 2020, ACMA launched a consultation on plans for area-wide apparatus licences for wireless broadband in the 24.7–27.5 GHz frequency range of the 26 GHz band; wireless broadband and FSS in the 27.5–29.5 GHz range; and FSS in the 29.5–30 GHz range. It is envisaged these licences would support private network and local WISP use-cases. ACMA is planning to make the spectrum at 24.7–25.1 GHz and 27.5–29.5 GHz available Australia-wide via an administrative process, with applications opening in October 2020 and licences available from December 2020. Licences for spectrum from 25.1 GHz to 27.5 GHz would be available via administrative process only after the conclusion of an auction of spectrum in this range covering key metropolitan and regional areas scheduled for Q1 2021. That administrative process is scheduled to begin in May 2021 with licences issued by the end of June 2021.
Belgium: In December 2019 BIPT launched a consultation on a draft bill and three draft royal decrees concerning among other things, the possibility of authorising local private networks using 4G or 5G in the 3800–4200 MHz band.
Brazil: An auction is expected in H1 2021 covering various bands with spectrum set aside for private networks: 2390–2400 MHz, 3700–3800 MHz, 27.5–27.9 GHz.
Chile: In November 2019 Chile passed a resolution designating spectrum from 3750 MHz to 3800 MHz for private 5G networks.
Croatia: Croatia is planning an auction of multiple spectrum bands. Due to the impact of COVID-19, the auction has been delayed from 2020 to H1 2021. It intends to auction at least 300 MHz in the 3410–3800 MHz range on a national basis, with remaining 90 MHz of spectrum potentially available for local or private networks.
Finland: Spectrum at 24.25–25.1 GHz is being reserved for the construction of local, private networks in the future.
France: At the end of March 2019, applications were closed for requests to run
5G trials at 26 GHz. The French regulator ARCEP accepted 11 schemes backed by public and private network operators. The various private network projects include inter alia deployment of a 5G network at the National Vélodrome in Saint-Quentin-enYvelines, a network at Lyon Part-Dieu station offering both public and private 5G services (the latter to enable station management and train telematics) and a network at The Grand Maritime Port of Le Havre for smart grid and logistics applications.
In May 2019, ARCEP opened up the possibility of wider private use of TDD spectrum at 2.6 GHz (2575–2615 MHz) for very-high-speed mobile networks (Air France KLM has already been trialling private LTE in this band for a number of years). Then in September, after a public consultation, it confirmed that it would be allocating the spectrum on a regional basis in order to improve broadband coverage for enterprises. Enterprises must express interest in using the spectrum, which will either be allocated (in the case of no competition for the spectrum) or ARCEP will determine a (possibly competitive) system of allocation. So far three applications have been accepted.
ARCEP has also opened the possibility of private network deployment in C-Band spectrum. In order to ensure organisations can get access to mobile services with sufficient coverage and performance, the terms of the licences for C-Band spectrum awarded in September 2020 included commitments to respond to reasonable requests from enterprise and public sector organisations for the supply of services, either through the supply of services on its own network or through sub-licensing of the frequency on a geographically limited basis.
Germany: In November 2019 Germany’s regulator, Bundesnetzagentur, opened up
applications for use of the 3700–3800 MHz band for local and regional 5G networks.
Stating that it wants Germany to be a pioneer in Industry 4.0, in addition to industrial users, the regulator anticipates the spectrum being leased by organisations in the agricultural and forestry sectors. Frequencies can be used immediately after allocation. The right to apply for spectrum derives from ownership or from another legal right to use the associated land, and fees are related to size of the geographic area covered by the licence, the amount of spectrum allocated and the duration.
The regulator has also been preparing a framework for the assignment of spectrum at 24.25–27.5 GHz for local applications. After consultation it is proposed the entire range be assigned on a technology and service neutral basis (starting with spectrum from 26.5-27.5 GHz). The new plans were opened up to a consultation which ended in August 2020. No limit is proposed for the geographical size of a local frequency assignment, although the licensee must have a plan for service availability throughout that area within a year. The results have not yet been published.
Hong Kong: In July 2019, the regulator OFCA announced that it would be making
400 MHz in the 27.95–28.35 GHz range available for Localised Wireless Broadband Licences (using 5G or other advanced mobile technologies) on a geographic-sharing basis. It opened up applications for assignment of the shared spectrum later the same month. GSA is aware of one active licence. OFCA has also stated plans to assign spectrum at 617–698 MHz and 703–803 MHz for indoor mobile services, with the spectrum available from 2021 at the earliest.
Japan: In December 2019, the Ministry of Internal Affairs and Communications began accepting applications for local 5G licences. The spectrum available spans 28.2–28.3 GHz and can be used within the applicant’s own building or on its own land to provide broadband fixed wireless services. National carriers are not eligible to apply, as the spectrum is not intended to supplement national carriers’ existing holdings. MIC also stated it would consider allocation of spectrum at 4.6–4.9 GHz and 28.2–29.1 GHz for local private services in the future. (In 2017, Japan also made the 1.9 GHz band, Band 39, previously used for PHS and DECT available for private LTE networks as a shared band.)
Malaysia: In January 2020, the MCMC stated that spectrum at 26–28 GHz will be assigned in two parts. Spectrum at 24.9–26.5 GHz will be tendered (beauty contest) to licensees on a national basis. Spectrum at 26.5–28.1 GHz will be assigned on a first-come, first-served basis for the deployment of local/private networks ‘for industrial and enterprise services and applications for, but not limited to, healthcare, ports, transportation, manufacturing, agriculture, public safety and smart city projects’. Proceedings have been delayed, however.
Netherlands: Spectrum at 3.5 GHz is already widely used for local and private network applications, although the government is reorganising the spectrum. It intends to make spectrum at 3500–3700 MHz available nationally from September 2022. Spectrum at 3400–3450 MHz and 3750–3800 MHz is then intended to be made available for local use from 2026. Spectrum at 3450–3500 MHz and 3700–3750 MHz is already used and currently protected for national security reasons. Netherlands’ Digital Connectivity Action Plan foresees the use of spectrum at 26 GHz either for a very large number of local permits or for shared use.
New Zealand: Spectrum at 2.5 GHz (2575–2620 MHz) is available in New Zealand for local or regional Managed Spectrum Park (private) licences (often for regional wireless broadband services). Eighty licences were awarded in 2009 after an initial round of applications. Subsequent licences have been made available on a first-come, first-served basis, with a number of licences awarded during 2019. Licences last for six years. There are limits on geographic coverage of licences held by any single organisation.
Norway: The Norwegian regulator Nkom is considering a joint assignment of various spectrum bands in 2021, two of which – the 2300–2400 MHz band and 3600 MHz – are under consideration for use for allocation to local/regional permits. In June 2020, it launched a consultation on plans to offer local/regional permits at 2300 GHz and 26 GHz.
Poland: In April 2019, Poland launched a consultation about the amount of spectrum to be made available at 3400–3800 MHz, with two options: a) 200 MHz in four lots of 50 MHz or b) 400 MHz (four national lots of 80 MHz plus 80 MHz for local use). In December, it announced a new consultation on the details of an auction of four lots at 80 MHz. Local use details had not been released at the time of writing.
Russia: In December 2019, Russia’s Deputy Minister of Digital Development, Telecommunications and Mass Communications stated that the government was preparing an auction of mmWave spectrum at 25.25–27.5 GHz (with six lots, four federal lots of 400 MHz and two regional lots of 250 and 400 MHz). Then in March 2020, SRCF published its decision that it would open up spectrum use at 24.25–24.65 GHz for an unlimited number of users for the purposes of creating private networks.
Russia SRFC is also reportedly planning to allocate chunks of spectrum at 400 MHz for private LTE networks.
Slovenia: In August 2020, Slovenia announced draft terms and conditions on plans for a multi-band spectrum covering various bands. A portion of the spectrum at 2300 MHz (2300–2320 MHz and 2390–2400 MHz) and at 3600 MHz (3400–3420 MHz) is expected to be set aside for local use (including for private use). The latest timetable envisages the completion of the auction before the end of 2020.
Sweden: PTS intends to enable local permits for the use of spectrum in the 3720–3800 MHz range. These will be awarded and managed through an administrative process. PTS initiated consultations on the demand for 5G frequencies in the 24.25–27.5 GHz bands and in December 2019, stated that it intended – as soon as possible – to allocate parts of the spectrum range for both local and large-scale 5G use. In its consultation, launched in April 2020, it proposed before the end of 2021 authorising the use of spectrum at 24.25–25.1 GHz for local 5G services, with licences valid to end 2025 and limited to indoor use.
UK: Following consultations, in July 2019, Ofcom announced plans to initiate spectrum sharing with localised licensing of key spectrum bands. Its aim is to open up use of the spectrum to private network operators such as enterprises and utilities. The spectrum that will be available through local licences includes:
• 3800–4200 MHz
• 1781.7–1785 MHz/1876.7–1880 MHz) (called 1800 MHz shared spectrum by Ofcom)
• 2390–2400 MHz (called 2300 MHz shared spectrum).
The spectrum is available on a coordinated first-come, first-served basis. Ofcom indicated that localised licenses can be applied for immediately
Ofcom has also decided to enable localised access to spectrum in the 26 GHz band (24.25–26.5 GHz) available on a sharedspectrum basis, but only for indoor use. (Spectrum in the 26.5–27.5 GHz range is used by the military. Ofcom will continue to review possible ways of making this spectrum available in the future.)
USA: There are more than 200 organisations holding PAL spectrum licences for CBRS deployments in the USA, including for private enterprise purposes, and more organisations intending to use General Authorized Access (GAA) spectrum. Initial commercial deployments of CBRS were given the go-ahead in September 2019. Full-blown commercial deployments were authorised in January 2020, initially only using General Authorized Access (GAA) spectrum. The auction of Priority Access CBRS spectrum at 3.5 GHz was completed in August 2020 enabling launch across all relevant spectrum ranges. The CBRS bands is expected to be used extensively for private mobile network deployments in the USA.
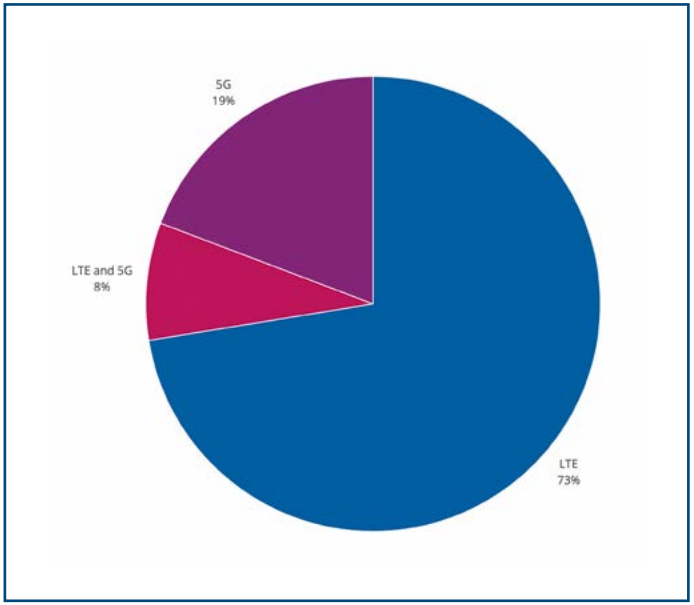
identified private network deployments, pilot and commercial
(base 156 networks)
Content on Shared Spectrum (C) GSA and reproduced courtesy of GSA.
For Further Information
Find out more about Shared Spectrum & Spectrum Sharing for Private 5G and Private LTE Networks:
Please Contact Us